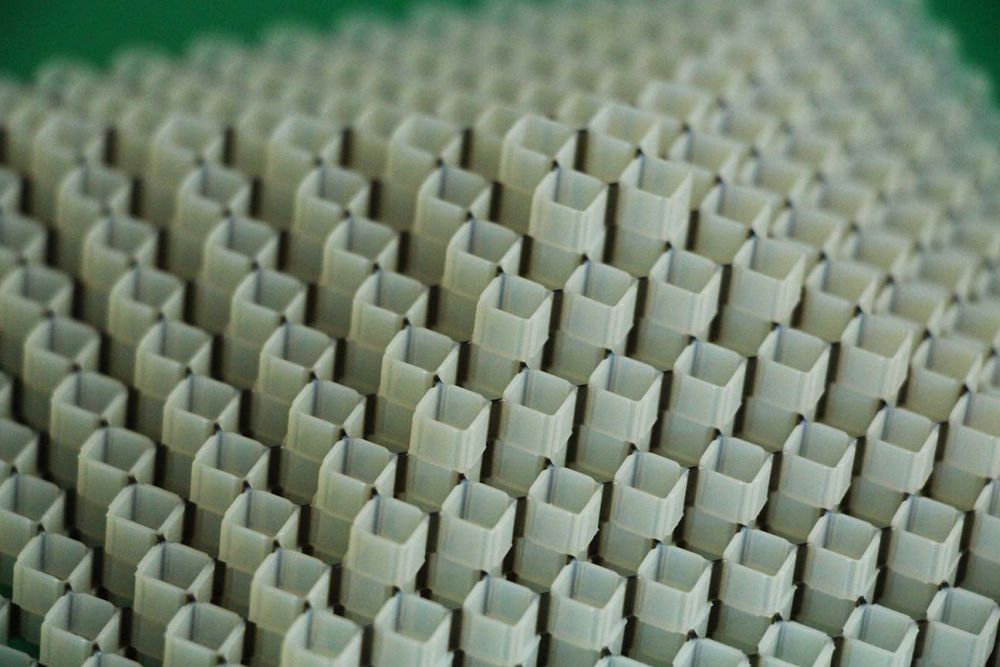
Mechanical metamaterials
Mechanical metamaterials are truly fascinating man-made materials that demonstrate extreme properties thanks to their sophisticated internal architecture. We work with heterogeneous mechanical metamaterials assembled from unit cells with various geometries. By harnessing local instability-driven transformations and integrating stimuli-responsive materials, we gain fine control over the performance of metamaterials and program their adaptive behavior. The field of mechanical metamaterials is relatively new and there is a long road ahead with many exciting discoveries to be made.
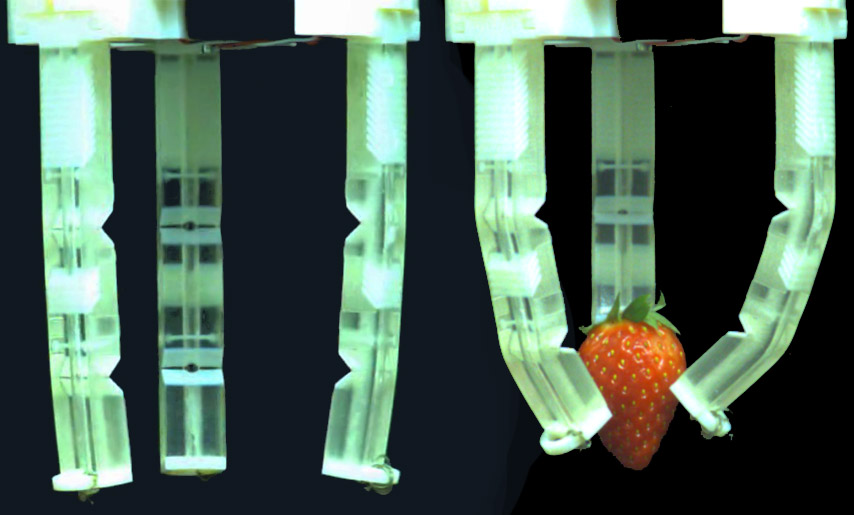
Soft Robotics
In contrast to their rigid counterparts, soft robots have infinite degrees of freedom. Actuation methods such as pneumatic, electric and magnetic can be used to trigger continuous morphing of the robot’s shape. Here we aim to develop methods for the programming of complex movement. In particular, we use a rational architecture that combines stiff and soft components. This reduces the complexity of the control system and improves the fidelity of the response.
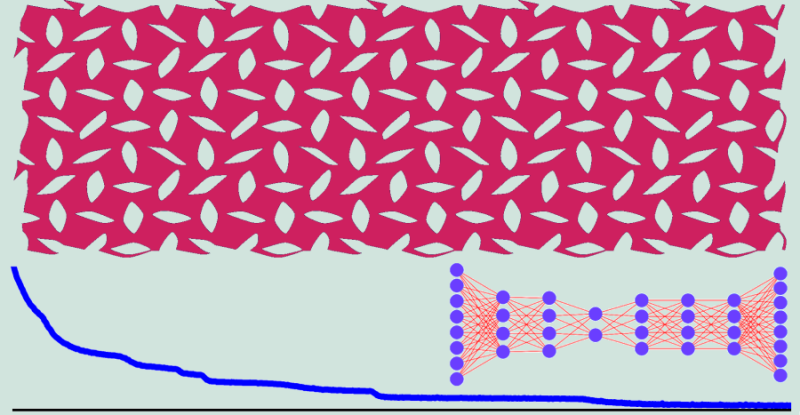
Machine Learning
We utilize machine learning (ML) to gain a deeper understanding of the relationship between a material’s microstructure and its properties. ML allows us to both predict the properties of engineered materials and solve the inverse problem of searching for configurations with a required behavior. Reinforced learning also helps us to “teach” metamaterials how to perform optimally.
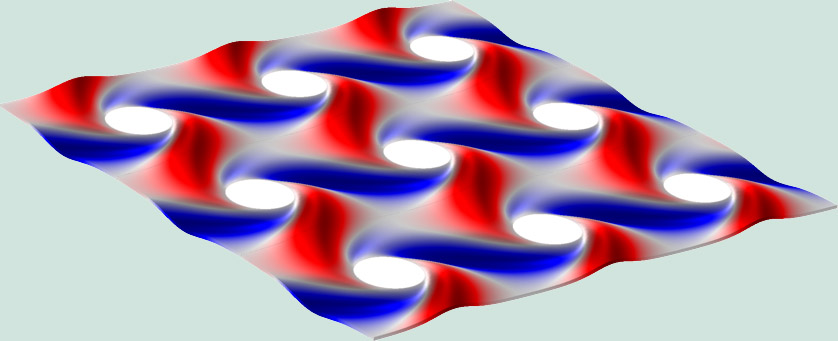
Elastic wave propagation
We study the propagation of elastic waves in soft composite structures. We endeavor to gain control over elastic waves using rational geometric designs. By applying external deformation and triggering local reconfigurations, we can open and close elastic bandgaps and realize more advanced wave-related phenomena.
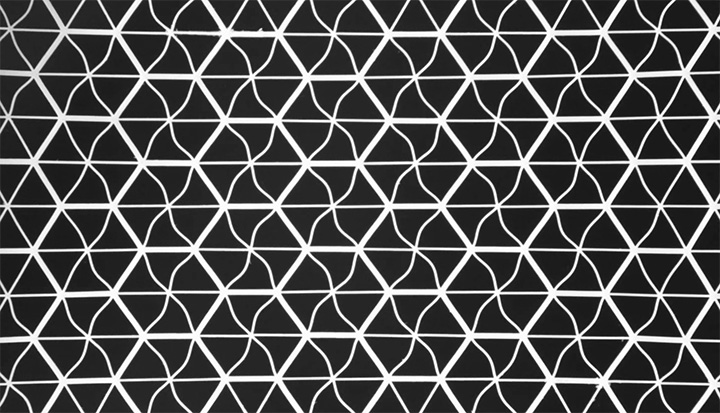
Instabilities and failure
Avoiding mechanical failure and loss of stability in materials and structures are important engineering considerations. Here we have adopted a novel new perspective on these phenomena. We are attempting to harness buckling and local failure as tools to alter and control the behavior of engineered materials. To this end, we study buckling and fracture using finite element modeling and experiments on 3D-printed composites.
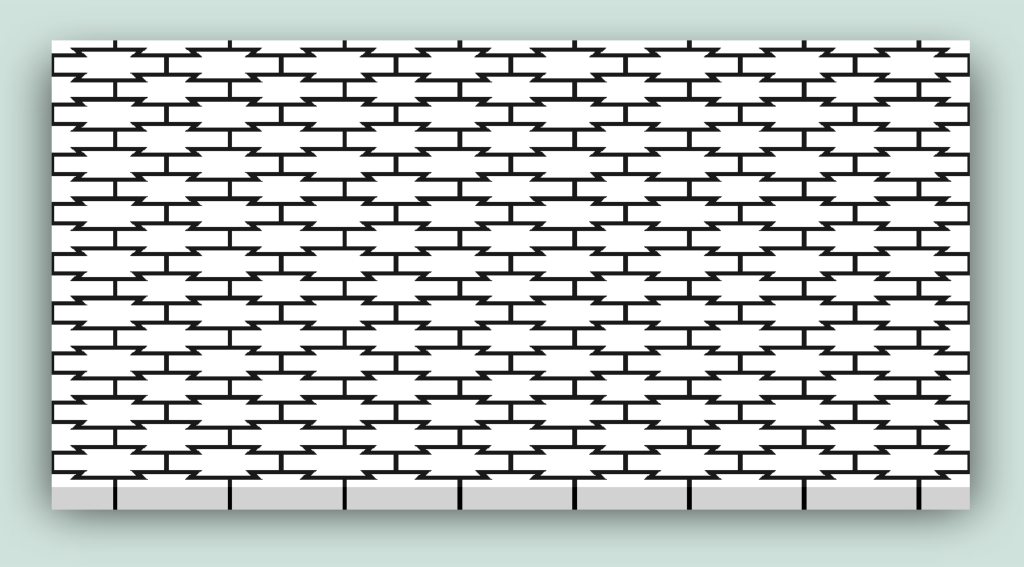
Bio-inspired materials
Over millions of years of evolution, nature has developed a multitude of materials that fascinate artists and engineers alike. We aim to learn from nature and mimic the structure of natural materials such as nacre using modern manufacturing techniques. This allows us to understand the mechanical mechanisms behind their extreme properties and to apply what we learn to engineer new and better materials.